On the morning of June 25, 2034, Sunspot NOAA-750 appeared to be unremarkable, sitting amongst the patchwork of other active regions on the Sun.
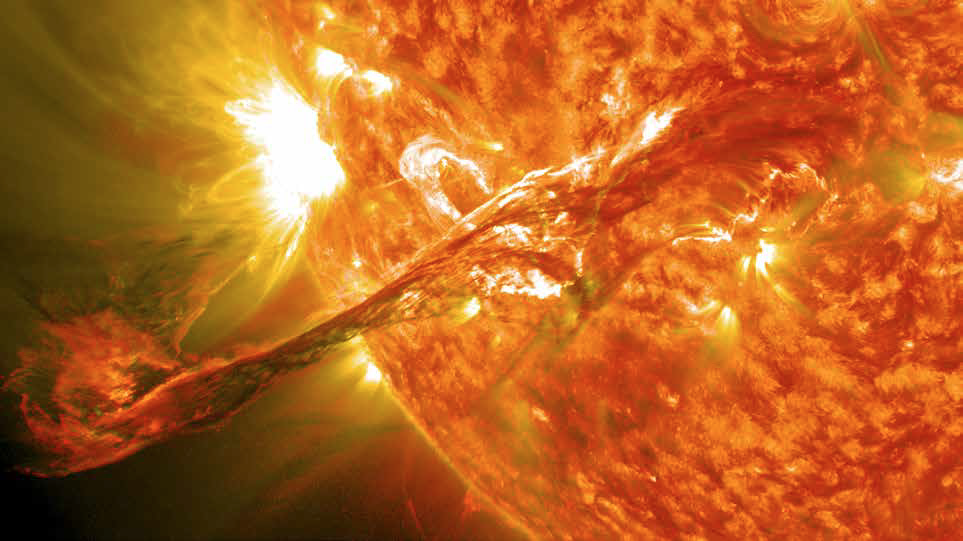
Figure 1. A solar flare, image courtesy of NASA.
That all changed at 10:00 a.m. UTC, when it suddenly erupted into the most intense solar flare ever observed — an X37 class storm that was many times more powerful than the previous record holder in 20031. Automated solar observatories set off NOAA alarms, triggering preparations for the dangerous proton storm and geomagnetic events that were expected in the hours and days ahead. (See Figure 1.)
Unfortunately, this flare had a rare characteristic with consequences that would soon turn deadly. Like the solar event in January 20052, there was a coincidental alignment of the solar flare’s position and the Sun’s spiraled magnetic field lines, which meant that this powerful flare had a perfect magnetic pipeline leading straight to Earth. A massive proton storm, accelerated to near-light speed with energies in excess of 100 MeV, followed this magnetic channel to impact the Earth a mere 11 minutes after the flare’s initial observation.
With no time to execute safe-mode shut downs, hundreds of satellites quickly became electrically charged and were rendered inoperative. Moreover, within hours, the increased solar irradiance caused perturbations and density changes in the upper atmosphere, resulting in rapid orbit degradations for thousands of orbiting objects. More than 50 military and Earth science satellites burned up before operators could regain control and execute corrective burns.
Also of grave concern was the cloud of orbital debris, carefully tracked for collision avoidance, whose pattern was suddenly altered on a global scale. All bets were off while computer models struggled to simultaneously reassess the trajectory of thousands of objects whose orbits were modified by the swelling atmosphere. Thankfully, no collisions occurred, although there were reports of an unusual “energy event” near the secretive U.S. Space Force Station, apparently indicating that the military crew had been forced to use some sort of proximity defense mechanism.
Through another stroke of good luck, there were no commercial or NASA cis-lunar flights in progress at the time of the flare, with one Virgin Deep Space shuttle having just returned to Earth. The NASA Deep Space Gateway (please see Figure 2), orbiting the Moon every 90 minutes, was also temporarily protected on the dark side of the Moon at the moment of the flare, and NASA alerted the crew of six using the lunar communications relay. The astronauts scrambled into the safety of the shielded center hub just in time, as they emerged into the sunlight and the full force of the proton storm that was, by then, well underway.
On the lunar surface, the Shackleton Crater Outpost was safe within the permanent shade of the crater rim, although the communications relays positioned above the rim quickly became inoperative. Two astronauts were outside of the outpost, installing a fifth solar panel in the perpetual sunlight of the crater’s southern edge. With communications cut off, they received no warming and continued to work for a full 30 minutes in direct sunlight while high energy protons hammered their bodies.
NASA flight surgeons estimated the two men had received a potentially lethal radiation dose of over 450 rem and were in dire need of immediate evacuation back to Earth. NASA was faced with a terrible decision: they could evacuate the dying men immediately, but this would risk exposing the spacecraft and the other healthy crew members to the flare’s inbound coronal mass ejection (CME), which was expected to arrive within 48 hours. In the end, NASA decided to wait out the CME before evacuating — a delay that ultimately cost the two astronauts their lives.
Meanwhile, back on Earth, the wave of hard x-rays from NOAA-750 had impacted the Earth’s ionosphere with no warning at all, followed by even more ionization from the powerful proton storm that arrived just minutes later. Ionospheric radio communications were completely blacked out and many radar systems beyond 40 degrees in latitude were rendered useless. Most critically, the ionospheric irregularities caused GPS signal delays and distortions so massive that even the high precision dual frequency GPS systems could not compensate. The world’s GPS systems essentially shut down.
In the air, the loss of GPS positioning was compounded by communications problems that disrupted the aircraft peer-to-peer separation network that had replaced the old centralized air traffic control centers. Suddenly, with almost no warning, thousands of aircraft were flying blind. For the most part, aircraft in flight did what they were meant to do: long haul drones activated their intense emergency strobes and shifted into tight holding patterns, while manned aircraft alerted their flight crews to take manual control. Despite these best efforts, in the foggy skies over Amsterdam, just 15 nautical miles from Schiphol airport, an inbound commercial flight collided with an unmanned air freighter with the loss of all 260 passengers and crew.
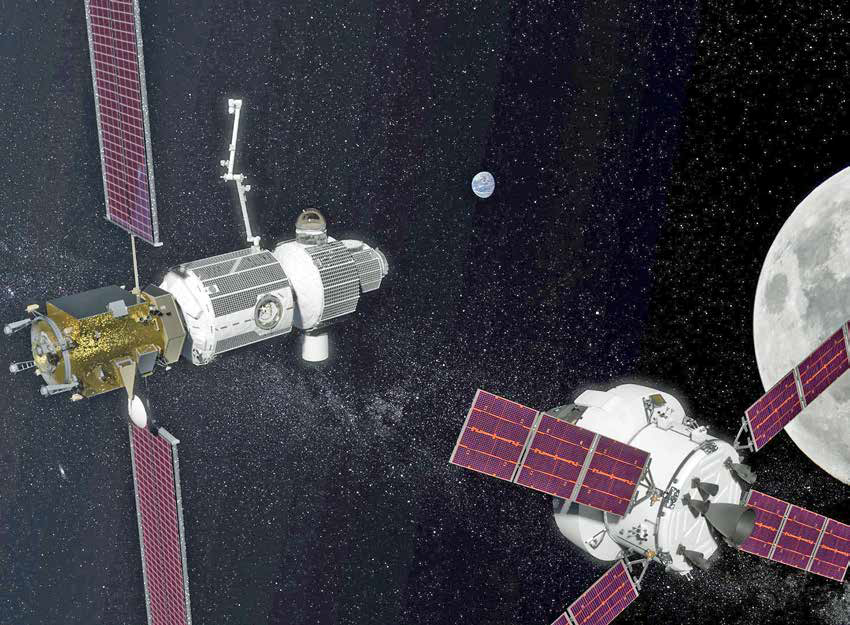
Figure 2. An artistic rendition of the proposed NASA Deep Space Gateway. Image is courtesy of NASA.
Back on the ground, millions of autonomous vehicles also responded to the complete loss of GPS positioning, and used their lidar systems to navigate to a safe stop. However, with every vehicle doing this simultaneously, the result was complete urban gridlock on a global scale. Emergency response vehicles, including police, ambulances and firetrucks, were unable to move for over 15 hours, resulting thousands of deaths and widespread civil unrest in cities around the world.
Fortunately, for reasons that heliophysicists are still trying to understand, the coronal mass ejection from NOAA-750 was far less severe than was predicted based on the intensity of the initial flare. Thankfully, the much feared “trillion dollar storm”3, with global power outages, did not materialize. Despite this lucky break, tens of thousands of deaths were attributed to the flare, while damage totaled over $690 billion, and there was also a sobering realization that things could have been much, much worse.
Back to the Future
This scenario is not just futuristic speculation; we have already experienced, studied and documented all of these dangers from extreme “space weather”, and scientists have even begun to agree on the probabilities of a Carrington-class storm hitting Earth within the next ten years: about 12 percent4.
“Carrington-class” refers to the massive solar storm that struck the Earth in 1859, which was studied by English astronomer Richard Carrington. The resulting distortions and fluctuations of the Earth’s magnetosphere were so intense that telegraph wires caught fire due to the large electric currents produced by the Faraday Effects of the geomagnetic storm. If the Carrington Event happened today, the result would be a global human catastrophe, thanks to our ever increasing reliance on satellites, delicate electronics, power grids, autonomous navigation, and information-packed radio communications.
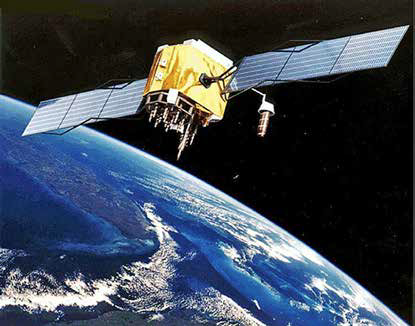
Figure 3. Artistic rendition of a GPS satellite. Image is courtesy of NASA.
In fact, a Carrington-sized solar eruption narrowly missed the Earth in July 2012. A mere seven days determined the difference between a benign solar storm directed out into the solar system and one directed Earthward, with devastating consequences3 5.
Even milder solar events have resulted in potentially dangerous situations on Earth and in space. For example, in November 2015, a relatively modest solar storm disabled the air traffic control systems in Sweden causing authorities to close off Swedish airspace for more than an hour6, while 25 years earlier, in March 1989, a solar storm took out a Hydro Québec generating station, leaving six million people without power for nine hours7.
There have also been multiple instances of degraded GPS navigation, such as the December 2005 solar flare that produced an immediate X-ray surge that disrupted satellite-to-ground communications and GPS navigation signals for more than ten minutes.
The military has long understood and studied these dangers. In May of 1967, a solar storm disrupted US military ionospheric communications over the polar cap. Given the jittery context of the Cold War, the U.S. Military interpreted this to be intentional radio jamming by the Soviet Union, and they shifted to high alert. Thankfully, the nascent Air Force space weather team was able to connect the disruption with an energetic solar event and deescalate the tense situation. More recently, in March 2002, during military operations in Afghanistan, a solar flare induced changes to the ionosphere that severely impacted military communications, and the resulting confusion led to a friendly-fire incident that left seven Americans dead8.
This is the context behind the ominously titled study that was prepared for the US Department of Joint Military Operations: “The Sun as a Non-State Actor: The Implications on Military Operations and Theater Security of a Catastrophic Space Weather Event”9. The report concludes that “a repeat of the historic Carrington Event of 1859 would devastate entire fleets of spacecraft and wipe out entire electrical grids”, and goes on to explain how world-renowned physicist Dr. Michio Kaku describes the worst-case scenario: “We’re talking about wiping out all satellite communications, all weather satellites, spy satellites, internet, GPS and blackening out most cities.”
Another problem for satellites in lower orbits is the change in atmospheric drag that solar flares can induce. The increased irradiance from flares causes low density layers of the upper atmosphere to rise so that spacecraft experience a stronger drag force. Even during routine periods of solar maxima, satellites may have to be maneuvered every two to three weeks to maintain their orbit10, but unpredictable solar events can produce rapid and very large increases in drag on satellites and alter their trajectory. For example, following the March 1989 solar storm, over 1,000 objects were “lost” due to sudden changes in their orbits, and it took the North American Aerospace Defense Command (NORAD) over a week to reacquire them and once again track their orbits with precision11.
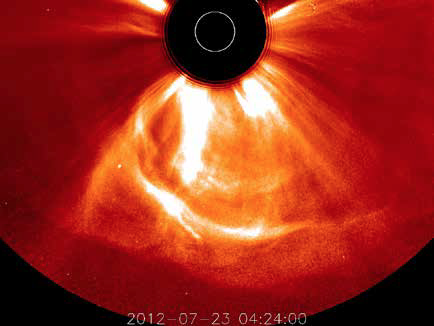
Figure 4. The “near miss” coronal mass ejection of July 2012, that left the sun at the unusually fast speeds of over 1,800 miles per second. Image credit: NASA / STEREO
As NASA moves ahead under the direction of the newly signed Space Policy Directive12, astronauts will soon leave the safety of Low Earth Orbit (LEO) for the first time in 45 years and return to the Moon and Mars soon thereafter. Once again, history provides us with a warning for the future: the solar storm of August 1972 thankfully occurred right in between the two manned missions of Apollo 16 and 17. Had the launch schedules been slightly different, Apollo astronauts could have been on the Moon at the time of the flare and suffered from radiation poisoning13.
More recently, in October 2003, a solar flare and subsequent coronal mass ejection forced the crew on board the International Space Station (ISS) to take shelter from the increased radiation (see Figure 6); meanwhile, far below the ISS, this same event caused diversions in polar region flight paths, degraded GPS performance, caused a Japanese satellite failure, and induced power outages in Europe and Africa8.
The U.S. government is aware of our increasing vulnerability to solar storms, as evidenced by recent government documents such as the 2017 National Space Weather Strategy, the National Space Weather Action Plan14 and the 2016 Presidential Executive Order for Coordinating Efforts to Prepare the Nation for Space Weather Events15. Among other things, these directives demand that NOAA, NASA, and other institutions, take immediate action to better protect our vital technical infrastructure from the next super storm, and — most critically — improve our ability to predict solar events to give us more time to prepare.
Some promising new research, centered on emerging the capabilities of artificial intelligence (AI), may help with the second part of that mandate: to predict and prepare.
Artificial Intelligence to the Rescue
A computer system is considered to demonstrate some level of artificial intelligence if it mimics cognitive functions that we would typically associate with biological intelligence, especially that of the human mind. Examples include strategy formulation in game play, complex image recognition, “learning,” and creative problem solving.
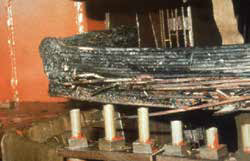
Figure 5. Permanent power grid transformer damage photo from 1989 solar storm. Photo credit: NASA
Deep Learning is a specific type of AI development that has received a great deal of attention due to its recent success in enabling computers to learn from vast amounts of data and subsequently make “intelligent” predictions, observations and decisions about that subject matter, even when the computer is shown new data that it has not seen before. Deep Learning systems accomplish this by using the mathematical concept of an artificial neural network, which loosely simulates information processing and adaptation patterns in a biological nervous system. This is a departure from the traditional approach to “teaching” a computer by manually writing a computer program that systematically encodes some aspect of human expertise.
The excitement is well placed, as there is growing evidence that something remarkable is taking shape within the intersection of high performance computing, massive data sets, and neural net algorithms. Problems which have been traditionally tackled with pensive coding, such as image classification and visual pattern recognition, have been overwhelmingly superseded with far more effective neural nets that often outperform the humans that trained them (See Figure 7).
This sea change is particularly relevant to NASA due to the petabytes of scientific data already within its archives, and because of the high affinity of new AI technologies to many space science problems that are top priorities to NASA, including space weather and heliophysics research.
Recognizing the potential value of AI to the space program, the NASA Frontier Development Lab (FDL) was launched in 2016 to become an applied artificial intelligence research accelerator that would combine the AI capabilities of NASA, academia, and private sector companies in support of NASA’s science priorities and mission goals. The program has been growing steadily, both in the scope of its AI investigations, as well as the range of commercial partners that are contributing with computing infrastructure and AI implementation experts.
The FDL 2018 research portfolio includes various AI projects grouped into seven major disciplines, including orbital debris and space weather. The FDL space weather teams will build from the progress made by researchers during the previous FDL 2017 cycle, in which scientists used AI Deep Learning tools, such as Google’s Tensorflow, to look for new ways to improve our ability to predict imminent solar events.
One of these teams focused on teaching Deep Learning neural nets to predict solar storms by analyzing high resolution images of the Sun16. The NASA Solar Dynamics Observatory (SDO) was a particularly useful source of training data for this work (See Figure 8). This satellite has been observing the Sun since 2010, and has been constantly streaming huge volumes of solar observation data back to Earth, including an uninterrupted sequence of HD-quality images of the Sun, taken every 12 seconds, year after year.
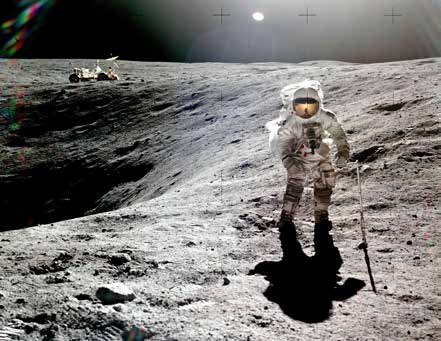
Figure 6. Apollo 16 astronauts on the Moon just before the August 1972 solar flare. Photo is courtesy of NASA.
The team also integrated information from other satellites, such as the GOES X-Ray observatory, to teach their neural network the difference in appearance between a “quiet Sun” and images of the Sun when flares were prevalent. The goal of the team was to produce an AI system that could estimate how strong a flare could be given the current state of the Sun.
Their work has produced several exciting indications of progress, such as the manner in which their neural network quickly learned to “pay attention” to active regions on the Sun. These are areas of intense and complex magnetic activity that can sometimes give rise to sunspots, and, more importantly, trigger solar eruptions such as solar flares and coronal mass ejections. As more data is fed into the AI system, the hope is that it will further refine its abilities – perhaps looking for a particular set of features within an active region, or specific combinations of active regions that together signal a potential solar flare may soon erupt.
The FDL team went on to generalize their AI framework to release an open source tool they call FlareNet16, which allows other heliophysicists to more easily build large collections of data to train new AI systems to predict other forms of solar phenomena. Using FlareNet, researchers are now able retrieve and combine archived data from two different SDO instruments: the Helioseismic and Magnetic Imager (which produces full-disk images of the Sun’s surface magnetic field) and the Atmospheric Imaging Assembly (which captures high definition images of the Sun more than eight channels spanning the UV and EUV spectrum). This can provide a much richer data set to train FlareNet, hopefully allowing it to tune into subtle hints of trouble before a solar storm erupts.
Meanwhile, a second FDL Space Weather team showed how an AI system, using Deep Learning technology, could predict variations of the Earth’s geomagnetic fields based on measurements of “solar wind”17. Solar wind is a stream of charged particles released from the upper atmosphere of the Sun, so establishing a predictive link to geomagnetic disturbances would be a big step forward, since these shifts in the Earth’s magnetic field can cause power grid failures and other infrastructure problems.
When they trained the AI system, it correctly deduced for itself that solar wind speed and proton density were important factors in making accurate predictions. In a particularly interesting twist, the AI system also determined that North-South component of the Earth’s geomagnetic field at low latitude monitoring stations — such as Guam, Hawaii, and Puerto Rico - are also important to predicting the intensity of a geomagnetic storm. The team realized that their AI program had tuned into a phenomena called “ring currents”; these are electric currents carried by charged particles trapped in the Earth’s magnetosphere and they have a significant effect on the electrodynamics of geomagnetic storms. The AI system was correctly learning about the complex physics of the Earth’s magnetic field. Perhaps, with more data and more training, this neural net might find new predictive factors that the scientists cannot explain, leading to new research and discoveries. At that point, it may be difficult to determine who is teaching who.
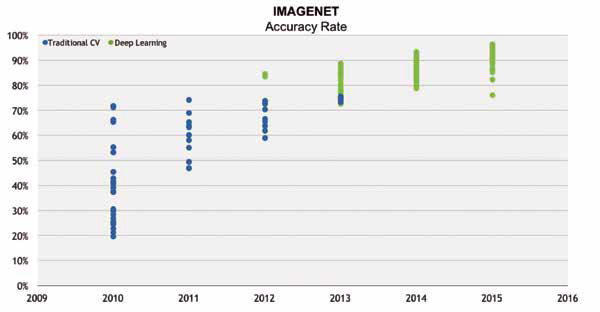
Figure 7. Progressive accuracy of the ImageNet competition results, showing that, since 2012, deep learning solutions have dominated the 10 million image classification task and now exceed human abilities. Chart is courtesy of NVIDIA Corp.
The space weather researchers have joined the FDL program because they believe that the application of artificial intelligence to heliophysics is a critical step in the taming the Sun and protecting the systems we all depend on. However, above and beyond the practicalities of making our technical infrastructure more resilient to solar events, there is our restless and relentless desire to understand the universe around us, to push out, explore, and learn what is beyond the horizon. The silicon chip was one of the key technologies that has allowed us to probe our solar system and have astronauts visit the Moon. Now, half a century later, artificial intelligence will help us to take the next step, to again explore beyond our home planet, not just to visit, but to stay.
References
[1] “SOHO Hotshots”, Solar and Heliopheric Observatory, NASA, 3 Nov. 2003, sohowww.nascom.nasa.gov/hotshots/2003_11_04/
[2] “A New Kind of Solar Storm”, NASA Science Beta, NASA, 10 Jun. 2005, science.nasa.gov/science-news/science-at-nasa/2005/10jun_newstorm
[3] “Near Miss: The Solar Superstorm of July 2012”, NASA Science Beta, NASA, 23 Jul. 2014, science.nasa.gov/science-news/science-at-nasa/2014/23jul_superstorm
[4] Riley, P. (2012), On the probability of occurrence of extreme space weather events, Space Weather, 10, S02012, doi:10.1029/2011SW000734.
[5] Baker, D. N., X. Li, A. Pulkkinen, C. M. Ngwira, M. L. Mays, A. B. Galvin, and K. D. C. Simunac (2013), “A major solar eruptive event in July 2012: Defining extreme space weather scenarios”, Space Weather, 11, 585–591, doi:10.1002/swe.20097.
[6] “Solar storm knocks out flight control systems in Sweden”, Phys.org News Service, 4 Nov. 2015, phys.org/news/2015-11-sweden-solar-flare-flight.html
[7] “A Super Solar Flare”, NASA Science Beta, NASA, 6 May 2006, science.nasa.gov/science-news/science-at-nasa/2008/06may_carringtonflare/
[8] “The trillion-dollar (solar) storm”, The Space Review, SpaceNews, 30 Oct. 2017, www.thespacereview.com/article/3358/1
[9] Major Brian W. Kabat, “The Sun as a Non-State Actor: The Implications on Military Operations and Theater Security of a Catastrophic Space Weather Event”, 03-05-2010, UNCLASSIFIED, Joint Military Operations Department.
[10] “Satellite Drag”, Space Weather Prediction Center, NOAA, www.swpc.noaa.gov/impacts/satellite-drag
[11] H. C. Koons and J. F. Fennell, “Space weather effects on communications satellites,” in URSI Radio Science Bulletin, vol. 2006, no. 316, pp. 27-41, March 2006.
[12] “New Space Policy Directive Calls for Human Expansion Across Solar System”, NASA Press Release, NASA, 11 Dec. 2017, www.nasa.gov/press-release/new-space-policy-directive-calls-for-human-expansion-across-solar-system
[13] “Sickening Solar Flares”, NASA Science Beta, NASA, 27 Jan. 2005, science.nasa.gov/science-news/science-at-nasa/2005/27jan_solarflares
[14] “National Space Weather Strategy And Action Plan Released”, Space Weather Prediction Center, NOAA, 9 Mar. 2017, www.swpc.noaa.gov/news/national-space-weather-strategy-and-action-plan-released
[15] “Executive Order -- Coordinating Efforts to Prepare the Nation for Space Weather Events”, The White House Office of the Press Secretary, The White House, 13 Oct. 2016, obamawhitehouse.archives.gov/the-press-office/2016/10/13/executive-order-coordinating-efforts-prepare-nation-space-weather-events
[16] Sean McGregor, Dattaraj Dhuri, Anamaria Berea, Andrés Muñoz-Jaramillo, “FlareNet: A Deep Learning Framework for Solar Phenomena Prediction”, Workshop on Deep Learning for Physical Sciences (DLPS 2017), NIPS 2017
[17] Mark C. M. Cheung, et al. Modeling Geomagnetic Variations using a Machine Learning Framework, in AGU 2017 Conference Proceedings. SM23A-2591, agu.confex.com/agu/fm17/meetingapp.cgi/Paper/281227
Graham Macintosh is an industry consultant in Applied A.I. for Space Science.